Marine toolbox
Common guides
Project Timing
Strategic management and delivering project studies
Services
Screening, ESIA, biodiversity action planning and monitoring
Issue Addressed
Duplicative advice and late integration of management actions
What is happening?
Why is it a problem?
How do we help to solve it?
Mitigation insights
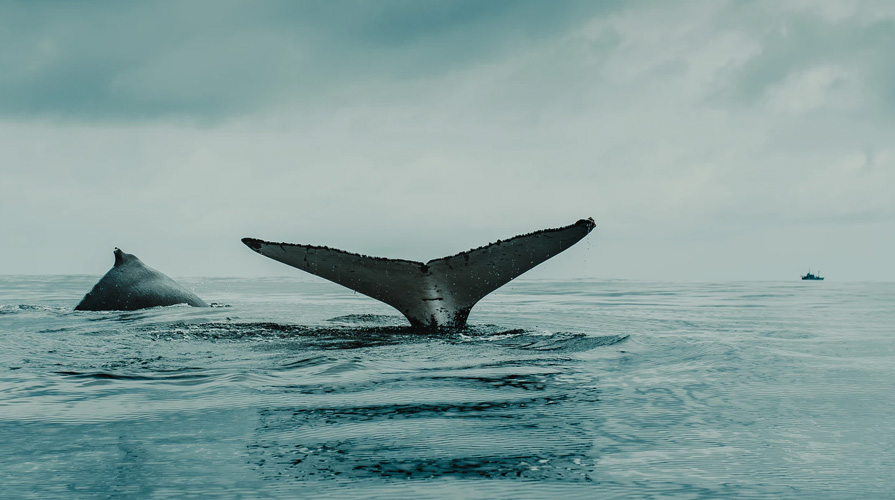
Ship strikes
Ship strikes pose a common risk to surface breathing marine wildlife which is associated with many marine development proposals – see e.g. Rockwood et al (2017; 2020) and Schoeman et al. (2020). Guidance on reducing the risk of ship strikes on marine mammals includes that produced by IMO and IWC, with actions such a routing, time-closures, detection, course, buffer distance, vessel speed restrictions and capacity building – e.g. IMO (2009), Ritter and Panigada (2014) and see various documents available at this IWC link. Such mitigation measures have been implemented in some sensitive areas for marine mammals, e.g., for North Atlantic right whales in eastern USA/Canada, Great Barrier Reef Marine Park, Glacier Bay, Santa Barbara, Peninsula Valdez, Hauraki Gulf, Mediterranean Sea etc. Research and guidance shows that avoidance through siting, detection, routing and/or time-closures are priority measures for addressing ship strikes on marine mammals – see e.g. Silber et al. (2012) and Frantzis et al. (2019). Vessel speed restrictions are also used as part of a mitigation strategy to reduce ship strike risk and are considered as being an important tool to minimise ship strike risks; and Vanderlaan and Taggart (2007), Conn and Silber (2013) and Laist et al. (2014), Leaper (2019) and Rockwood et al. (2020) provide further information on the role of such measures for marine mammals. Speed restrictions that are implemented to minimise impacts on marine mammals are generally 10 knots and 12 knots, with the former being most implemented. However, Kelley et al. (2020) reported that for their analysis of large vessels that a speed restriction of 10 knots will provide only small reductions in the probability of lethal ship strikes on North Atlantic right whales. They again reported on the need to implement good avoidance measures to safeguard marine mammals from strikes with large vessels. Kelley et al. (2020) reported on the greater effectiveness of speed restrictions and visual watches to promote evasion for small vessels.
Hazel et al. (2007) report that very low vessel speeds may be required for sea turtles in comparison to the restrictions commonly implemented to minimise impacts on marine mammals from their study of green turtles in Moreton Bay, Australia. They report that vessel operators cannot rely on turtles to actively avoid being struck by the vessel if it exceeds just over 2 knots. Examples of low speed and routing restrictions for sea turtles can be found in Zakynthos National Marine Park in Greece where navigation is either prohibited or vessel speeds are restricted to 6 knots. In Moreton Bay Marine Park, go-slow areas are defined for sea turtles (and dugong) that limits speed to 10 knots for vessels over 8 m, and for travel in shallow waters, to be off-plane or in displacement modes.
References
Conn, P.B and Silber, G.K. 2013. Vessel speed restrictions reduce risk of collision‐related mortality for North Atlantic right whales. Ecosphere 4(4):43.
Frantzis A., Leaper R., Alexiadou P., Prospathopoulos A. and Lekkas D. 2019. Shipping routes through core habitat of endangered sperm whales along the Hellenic Trench, Greece: Can we reduce collision risks? PLOS ONE 14(2): e0212016.
Hazel, J., Lawler, I., Marsh, H. and Robson, S. 2007. Vessel speed increases collision risk for the green turtle Chelonia mydas. Endangered Species Research. 3. 105-113. 10.3354/esr003105.
IMO. 2009. Guidance documents for minimising the risk of ship strikes with cetaceans. MEPC.1/Circ.674. 31 July 2009.
Kelley, D. E, Vlasic, J.P and Brillant, S.W. 2020. Assessing the lethality of ship strikes on whales using simple biophysical models. Marine Mammal Science. 12 October 2020.
Leaper, R. 2019. The role of slower vessel speeds in reducing greenhouse gas emissions, underwater noise and collision risk to whales. Front Mar Sci 6:1−8.
Laist, D. Knowlton, A. and Pendleton, D. 2014. Effectiveness of mandatory vessel speed limits for protected North Atlantic right whales. Endanger. Species Res. 23.
Rockwood R.C., Calambokidis J., Jahncke J. 2017. High mortality of blue, humpback and fin whales from modeling of vessel collisions on the U.S. West Coast suggests population impacts and insufficient protection. PLoS ONE 12(8): e0183052.
Rockwood, R.C, AdamS, J., Silber, G. and Jahncke, J. 2020. Estimating effectiveness of speed reduction measures for decreasing whale-strike mortality in a high-risk region. Endang Species Res. Vol. 43: 145–166, 2020.
Ritter, F. and Panigada, S. 2014. IWC Guidance for Cruise Line Operators to Minimise Risk of Collisions with Cetaceans.
Schoeman Renée P., Patterson-Abrolat C., and Plön S 2020. A Global Review of Vessel Collisions With Marine Animals. Frontiers in Marine Science. Vol. 7, 2020.
Silber, G.K., Vanderlaan, A.S.M, Arceredillo, A.T., Johnson, L., Taggart , C.T., Brown, M.W., Bettridge, S., Sagarminaga, R. 2012. The role of the International Maritime Organization in reducing vessel threat to whales: Process, options, action and effectiveness. Marine Policy 36, 1221–1233.
Vanderlaan, A. and Taggart, C. 2007. Vessel collisions with whales: The probability of lethal injury based on vessel speed. Marine Mammal Science. 23. 144 – 156.
Disturbance to sea turtle nesting beaches
We’re often asked to consider footprint impacts in areas where sea turtles nest. Once a site is confirmed in a nesting area, options are generally limited to nesting season avoidance, protecting nests in-situ for the entire incubation period or translocation of eggs. However, we rarely see avoidance proposed when we review mitigation strategies for projects. In situ nest protection can be seen as restrictive. However, the complications associated with other mitigation measures are not always appreciated by developers or their advisors at an early stage or whenever it is proposed within the project cycle. Translocation will not be successful unless done in an appropriate way and it requires intensive monitoring. Translocation of sea turtle eggs after two hours from laying requires special care if successful hatching is to occur as reported upon by Mortimer (1999), which relates to the potential for movement induced embryo mortality after this time. Some standard guidance recommends that eggs be relocated within 12 hours, which is based on historic research that showed much lower levels of hatching success after this time, e.g. Limpus et al. (1979) and Parmenter (1980). Parmenter (1980) and Miller (1999) recommended that eggs be left in situ for at least 25 days if not moved within 2 hours to limit the potential for mortality, which relates to the time for the development of the embryo and its membranes – see Miller et al. (2003). Ahles and Milton (2015) have reviewed the success of egg relocation over certain incubation periods and have identified increased mortality after 12 hours of movement. All of the above research can therefore be used to determine good practice approaches for translocation of sea turtle eggs or determining the need for in situ nest protection. Guaranteeing successful outcomes, is however, complicated requiring careful planning and delivery; and is often costly to deliver in practice (e.g. it may require ongoing monitoring at night). Understanding such issues is important when providing good mitigation advice, especially when appreciating the value of avoidance which can be much easier and cost effective to achieve. Avoidance of impacts should always be preferred and appreciating the consequence of other measures is important for promoting acceptance of the best approach.
References
Ahles, N. & Milton, S. 2015. Mid-incubation relocation and embryonic survival in loggerhead sea turtle eggs. The Journal of Wildlife Management. 80.
Limpus, C.J, Baker, V. and Miller, J. D. 1979. Movement Induced Mortality of Loggerhead Eggs. Herpetologica, Vol. 35, No. 4 (Dec., 1979), pp. 335-338.
Miller, J.D. 1999. Determining Clutch Size and Hatching Success. In: K. L. Eckert, K. A. Bjorndal, F. A. Abreu-Grobois, M. Donnelly (eds). Research and Management Techniques for the Conservation of Sea Turtles. IUCN/SSC Marine Turtle Specialist Group Publication No. 4, 1999.
Miller J, Limpus C, Godfrey M. 2003. Nest site selection, oviposition, eggs, development, hatching, and emergence of loggerhead sea turtles. In: Bolten A, Witherington B (eds) Loggerhead sea turtles. Smithsonian Institution Press, Washington, DC, p 125–143
Mortimer, J. 1999. Reducing Threats to Eggs and Hatchlings: Hatcheries. In: K. L. Eckert, K. A. Bjorndal, F. A. Abreu-Grobois, M. Donnelly (eds). Research and Management Techniques for the Conservation of Sea Turtles. IUCN/SSC Marine Turtle Specialist Group Publication No. 4, 1999.
Parmenter, C.J. 1980. Incubation of the Eggs of the Green Sea Turtle, Chelonia Mydas, in Torres Strait, Australia: the Effect of Movement on Hatchability. Australian Wildlife Research 7(3), 487 – 491
Underwater sound impacts and sea turtles
Impacts from underwater sound are something we are commonly asked to consider. Most practitioners are aware of guidance for minimising the risk of injury to marine mammals (e.g. UK JNCC – see piling noise, explosives and geophysical surveys; Brazil IBAMA (2018) (Vilardo et al., 2018); US BOEM (2012); Canada DFO (2007)). Often, I see measures in such guidance being used to mitigate impacts on sea turtles; and this is not always appropriate. Sarah Nelms et al (2016) provide a great review of the relevance of existing underwater sound mitigation guidelines for seismic activities for sea turtles. Importantly, they conclude that avoidance (including time-closures) are likely the best form of mitigation given the uncertainties and limitations that exist. Examples of national guidance for such avoidance exist in Brazil and Gabon (National Parks); and can be implemented at a project level. Also, it’s sometimes forgotten that guidance focuses on injury; and developing mitigation for behavioural impacts on marine wildlife should always be considered additionally as appropriate. This is often limited by uncertainties and therefore may require precautionary mitigation to be considered and adopted as early as possible with avoidance measures being key.
References
S.E. Nelms, W.E.D. Piniak, C.R. Weir, B.J. Godley, 2016. Seismic surveys and marine turtles: an underestimated global threat? Biol. Conserv., 193, pp. 49-65
Vilardo, C., Barbosa, A., Viana, M., Farrenberg, M., Campos, F. and Cirne, P. 2018. Guidelines – Marine Biota Monitoring in Seismic Surveys. 10.13140/RG.2.2.24471.01449.
Artificial lighting
Light Pollution is an issue that we are frequently asked to consider and mitigate for marine wildlife. The Florida Marine Institute’s guidelines produced by Witherington and Martin (2003) have long been a key reference when considering impacts on sea turtles. A recent reference point are the Light Pollution Guidelines drafted by Kellie Pendoley et al. and published as final guidance by the Australian Department of the Environment and Energy in January 2020. This recent guidance focuses on sea turtles and seabirds that are often most impacted by light in the coastal and marine environment. This is a great common resource for consultants, developers and conservationists dealing with artificial lighting issues. This guidance was adopted by the Convention on Migratory Species signatory states in February 2020.
References
Witherington, B. and Martin, R.E. 2003. Understanding, Assessing, and Resolving Light-Pollution Problems on Sea Turtle Nesting Beaches. Florida Marine Research Institute. Technical Reports.
Department of the Environment and Energy. 2020. National Light Pollution Guidelines for Wildlife Including marine turtles, seabirds and migratory shorebirds.
Entrapment of sea turtles in seismic survey equipment
Contributions from Caroline Weir
Offshore seismic surveys can lead to unintended sea turtle mortality as a result of entrapment in towed seismic gear. This includes risks from entrapment in streamer tail buoys ( large float attached to the end of each seismic cable used to monitor the location of the cables) and also potentially in some dilt floats. Mortality risks mainly relate to tail buoys with dual tow points and an open undercarriage. Such risks can be reduced through adopting appropriate design mitigation. Ketos Ecology (2009) have considered how to mitigate impacts associated with these risks. Their review has identified design avoidance options, including a preference for the use of alternative ‘turtle friendly’ designs – meaning those buoys with designs where there is no possibility of turtles becoming trapped, i.e. no subsurface gaps and appropriately positioned tow chains. Other alternative options include the fitting of ‘exclusion turtle guards’ and ‘deflector turtle guards’ to existing tail buoys that provide a risk of entrapment and/or the implementation of other measures, such as, re-locating tow points, chain placement and fitting Norwegian buoys ahead of each tail buoy. However, the effectiveness of these alternative measures is uncertain; and Ketos Ecology (2009) reported on some mortalities that occurred even with guards fitted to existing tail buoys that posed a risk for entrapment. Therefore, the key recommendation made by Ketos Ecology (2009) is for ‘turtle friendly’ designs to be used to avoid risks.
References
Ketos Ecology. 2009. Turtle guards: a method to reduce the marine turtle mortality occurring in certain seismic survey equipment. Ketos Ecol. Rep. 1–14.
Seagrass restoration
With contributions from Mark Fonseca, Ph.D., Vice President Science, CSA Ocean Sciences, Inc.
Seagrass restoration techniques have been trialled over many years. Early reports on restoration include the seminal paper for eelgrass restoration in the U.S. dating back to the mid-twentieth century (Addy, 1947). Fonseca (2011) reported that many of the lessons learned from Addy’s work are still relevant. Over time, research and guidance have continued to build worldwide that describes the promises and pitfalls of seagrass restoration (see references below).
Successful seagrass restoration leads to unique marine biodiversity benefits if undertaken successfully. However, the ability to ensure seagrass restoration success remains inherently limited. Recognising the sources of these limitations is important for development projects when considering restoration mitigation where impacts on seagrass habitat may occur, for restoration offsetting and to achieve additional conservation outcomes. Fonseca et al. (1998) found that the successful establishment of seagrass cover occurred in <50 % of projects they reviewed at that time. They also reported that for most projects they reviewed at that time that most seagrass failed to achieve the goal of 1:1 habitat replacement. Bayraktarov et al. (2016) found a 38 % success rate in a global assessment of marine restoration. Similarly, Rezek et al. (2019) assessed 33 seagrass mitigation projects ranging from 3 to 32 years old and found that 88 % produced persistent seagrass, but that seagrass coverage were 37 % lower than reference beds, although comparison to the reference beds was problematic. In the U.S., success thresholds (usually defined as percent survival of individual plantings) in permitting are often set at 70-90 %. Based on the success of restoration, Fonseca (2011) reported that ‘conservation of seagrass remains a more reasonable alternative than restoration’. This highlights the difficulty in relying on restoration for fully mitigating impacts on seagrass habitats and the importance of implementing earlier steps in the mitigation hierarchy (avoid and minimise). Fonseca et al. (1998) also considered functional equivalence, which is an important principle for restoration and offsetting. They reported that whilst this development of an environment that is of comparable value may occur, there is still a measurable period until systems attain full function and compensation for those interim lost services is needed (Fonseca et al., 2000).
Examples of guidelines for seagrass restoration include Phillips (1980), Fonseca et al. (1998), Merkel et al (1992), Turner et al. (2006), van Katwijk et al. (2009), and UNEP-Nairobi Convention/ WIOMSA (2020). van Katwijk et al. (2015) provided a global review of seagrass restoration to identify characteristics that promote restoration success. From such literature, important aspects for successful restoration include appropriate site selection (i.e. restoration where seagrass exist and can recover and proximity to donor beds), understanding local conditions (biophysical conditions and species being transplanted), scale of restoration, presence of existing threats and using appropriate techniques related to local conditions. van Katwijk et al. (2015) also reported on the importance of restoration at larger scales to promote success.
Transplanting techniques that have been adopted globally are numerous and most work well. The techniques used, include for example, plug methods (transplanting of sediment cores containing seagrass), staple method (removal of plants from the sediment and attaching to metal or wood staples for planting elsewhere), peat pot method (plugging and planting in peat pots), use of whole sods and even for some species, attachment to sand-filled hessian bags. However, these techniques have a range of success and are costly to implement (although monitoring programs compliant with regulatory or financing requirements may be similarly costly).
Matching the technique to the growth strategy of the seagrass species is an important step for successful colonisation. In addition to transplanting, spreading of seagrass seeds has been developed in more recent times; and this has been proven to be a successful method for restoring a limited number of seagrass species around the world. These techniques tend to be less labour intensive and at lower cost, but also mimics a natural process that relies on a high ratio of seed production to seafloor colonisation over time; meaning that sowing of seeds can have especially variable success (but see Orth et al., 2012). This requires the collection of ripe, seed laden reproductive shoots. Deployment of seeds globally has used various techniques including spreading by hand at the water surface, the use of seed buoys, the planting of seeds in coconut matting and the use of hessian bags for snagging of drifting seed (Unsworth et al., 2019). Again, understanding the life history and reproductive strategies of the seagrass species to be restored is an important aspect of successful restoration.
Therefore, when addressing development impacts the challenges that exist for effective seagrass restoration need to be appropriately considered, especially with respect to achieving No Net Loss and Net Gain. The goals of restoration need to be clearly defined and limitations understood; and careful planning and management is needed to drive success. There is an ongoing need to explore alternative restoration strategies, including creation of mitigation banks where greatest success may be possible through consolidation of many small projects and so mitigating risk. Additionally, ensuring that mitigation measures are treated holistically – for instance addressing physical environmental factors like wave climate and water quality that may promote greater success bear further consideration. Although seagrass restoration has occurred over many years, fully mitigating losses through restoration is difficult and creating gains is not guaranteed. Acknowledging the complexity in seagrass restoration provides an important context for decision-making, especially at early project stages when there is greater opportunity to implement other mitigation options. This includes prioritising avoidance of impacts on seagrass beds where degradation and loss may occur, in line with the proper application of the mitigation hierarchy. This is especially important due to the important, almost irreplaceable ecosystem functions that seagrass beds provide. Nonetheless, if undertaken successfully, seagrass restoration forms an important component of the mitigation toolbox and in creating additional conservation outcomes.
References
Addy, C.E. 1947. Eel grass planting guide. Maryland conservationist 24:16–17.
Bayraktarov, E., M.I. Saunders, S. Abdullah, M. Mills, J. Beher, H.P. Possingham, P.J. Mimby, C.E. Lovelock. 2016. The cost and feasibility of marine coastal restoration. Ecological Applications 26(4):1055-1074.
Fonseca, M.S. 2011. Addy revisited: what has changed with seagrass restoration in 64 years? Ecol. Restor. 29, 73–81.
Fonseca, M. S., Kenworthy, W. J., and Thayer, G. W. 1998. Guidelines for the Conservation and Restoration of Seagrasses in the United States and Adjacent Waters. Silver Spring, MD: NOAA/National Centers for Coastal Ocean Science.
Fonseca, M.S., B.E. Julius, and W.J. Kenworthy. 2000. Integrating biology and economics in seagrass restoration: how much is enough and why? Ecol. Engineer. 15:227-237.
Merkel, K.W. 1992. A field manual of transplantation techniques for the restoration of Pacific coast eel grass meadows.Pacific Southwest Biological Services, Inc. National City, CA. 42pp.
Phillips, R.C. 1980. Planting Guidelines for Seagrass. U. S. Army Corps of Engineers, Coastal Engineering Aid, U.S. Army Corps of Engineers, Waterways Experiment Station,Vicksburg, MS.
Orth R.J., Moore K.A., Marion S.R., Wilcox D.J., Parrish D.B. 2012. Seed addition facilitates eelgrass recovery in a coastal bay system. Mar Ecol Prog Ser 2012;448:177–195
Turner, S., A.M. Schwarz. 2006. Management and conservation of seagrass in New Zealand: an introduction. New Zealand Department of Conservation, Science fo Conservation series.
Rezek, R.J., B.T. Furman, R.P. Jung, M.O. Hall, and S.S. Bel. 2019. Long-term performance of seagrass restoration projects in Florida, USA. Nature Scientific Reports. 2019) 9:15514
UNEP-Nairobi Convention/WIOMSA 2020. Guidelines for Seagrass Ecosystem Restoration in the Western Indian Ocean Region. UNEP, Nairobi, 63 pp.
Unsworth RKF, Bertelli CM, Cullen-Unsworth LC, Esteban N, Jones BL, Lilley R, Lowe C, Nuuttila HK and Rees SC (2019) Sowing the Seeds of Seagrass Recovery Using Hessian Bags. Front. Ecol. Evol. 7:311.
van Katwijk, M.M., Bos, A.R., de Jonge, V.N., Hanssen, L.S.A.M., Hermus, D.C.R., and de Jong, D.J. 2009. Guidelines for seagrass restoration: Importance of habitat selection and donor population, spreading of risks, and ecosystem engineering effects. Marine Pollution Bulletin 58,179–188
van Katwijk, M. M., Thorhaug, A., Marba, N., Orth, R. J., Duarte, C. M., Kendrick, G. A., et al. 2015. Global analysis of seagrass restoration: the importance of large-scale planting. J. Appl. Ecol. 53, 567–578. doi: 10.1111/1365-2664.12562